- Carbon sinks are places and/or products that sequester and store carbon.
- The ocean, which is by far the largest carbon reservoir and sink, stores carbon in several different forms. The most abundant form is as dissolved inorganic carbon.
- Currently, India’s goal under the Paris Agreement is set at creating an additional 2.5–3 bilion tonnes of carbon dioxide equivalent through additional forest and tree cover by 2030.
Carbon sinks are places and/or products that sequester and store carbon as organic or inorganic compounds for different periods of time. Essentially, anything that absorbs more carbon than it emits into the atmosphere through natural or artificial processes can be considered a carbon sink.
In the pre-industrial era, carbon emitted into the atmosphere was usually balanced – on a global scale – by the accumulation of carbon in terrestrial and oceanic systems known as carbon sinks.
But since humans began using fossil fuels to power industrialisation in the 1960s, carbon dioxide (CO2) levels in the atmosphere have risen by nearly 100 ppm (parts per million) to reach a record high of 400 ppm due to rapidly multiplying carbon sources. Carbon sources are places and processes that release carbon into the atmosphere (mostly in the form of carbon dioxide, particulate carbon, and methane). These include burning fossil fuels, intensive agriculture, and raising livestock, which are leading to large scale climate changes including global warming and extreme weather phenomena.
What are natural and artificial carbon sinks?
Natural carbon sinks can be terrestrial – soil, forests, and grasslands – or aquatic, namely, the ocean. Currently, the oceans are the largest carbon reservoirs in the world.
Artificial carbon sinks, meanwhile, have been proposed to combat the rising levels of carbon in the atmosphere. One such idea is to convert rapidly growing urban areas into carbon sinks by using engineered timber instead of cement, steel, and other such materials. This approach aims to not only create new carbon sinks, but to also simultaneously lower carbon emissions by reducing the demand for cement, since cement manufacture currently contributes to 8% of global CO2 emissions.
Another idea for an artificial carbon sink is to capture CO2 directly from air or point sources (such as factories/industrial areas) and create a concentrated stream of CO2 for use in the production of carbon neutral fuels or carbon sequestration using various methods. Carbon sequestration methods can involve injecting CO2 into the ocean or into geologic formations.
Since 1996, deep saline aquifers in the Sleipner natural gas field in Norway have been used as storage areas for CO2 by the Norwegian oil company Equinor, which initiated the project to avoid paying carbon taxes. However, problems with pumping CO2 into the ocean are many, and recent investigations into leaks in the Sleipner storage sites indicate that geologic storage solutions for CO2 are also quite problematic.
Mineral sequestration of CO2 is another carbon capture method that is being explored to create carbon sinks. In this, the carbon is trapped in the form of solid carbonate compounds in sandstone and basalt. Ongoing research projects are also investigating the potential of using mining waste for CO2 sequestration.
How do oceans act as carbon sinks?
The ocean, which is by far the largest carbon reservoir and sink, stores carbon in several different forms.
The most abundant form is as dissolved inorganic carbon (DIC). This entered the seas over millennia through sea water interacting with sediments, land weathering, outgassing from the Earth’s interior (for example, through volcanic vents), and gas exchange with the atmosphere. The CO2 usually dissolves in seawater, where it exists as CO2, bicarbonate ions (HCO3–), and carbonate ions (CO3–).
The intermediate and deep ocean is estimated to be the largest of the five carbon reservoirs in the ocean, the other four being the surface ocean, marine biota, dissolved organic carbon (DOC) and sea surface sediments.
Oceans also act as carbon sinks due to that actions of biological carbon pumps, which occur mainly in the surface layers of the ocean. Phytoplankton convert CO2 from the air and dissolved CO2 in seawater into biomass through photosynthesis. About 25% of this is particulate organic carbon (POC) that sinks through the water column. Most of the sinking POC is remineralised by microbes and less than 1% of the POC reaches the ocean floor by sedimentation to contribute to the carbon sink in the ocean floor.
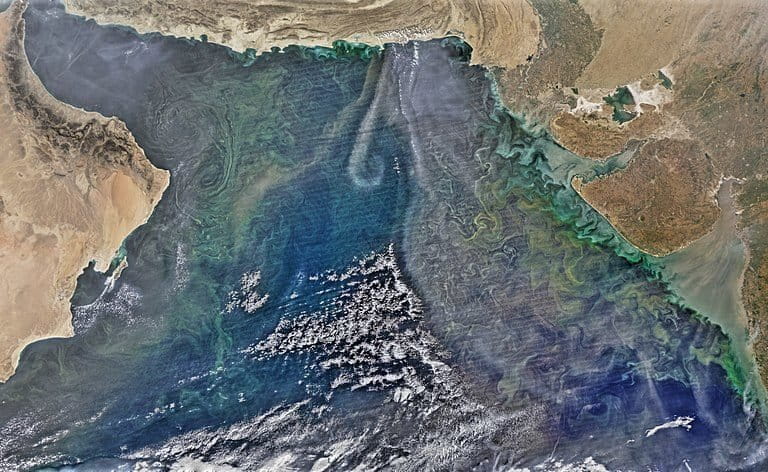
Marine biota also produce DOC or dissolved organic carbon, which differs from POC in particle size (DOC particles are considerable smaller than POC particles). Due to their small size, DOC particles do not sink, but remain suspended in surface waters, where they feed the classical food chain by serving as nutrients for microbes at the lowest trophic levels. In addition to DOC and POC, calcium carbonate in the form of calcite or aragonite, formed by shell/skeleton producing marine organisms, is an important biotic carbon sink.
In 2019, it was estimated that the oceans absorbed 30% of the atmospheric CO2 emissions caused by human activity between 1994 and 2007. A recent study in 2020 further shows that the oceans continue to act as a sink for ~25% of anthropogenic CO2 emissions.
However, this increased CO2 uptake by oceans may not last, as they are not infinite carbon sinks. As more CO2 enters the ocean, the changes in the ratios of dissolved CO2, HCO3–, and CO3– ions affect the buffering capacity of the seawater. This means that as the DIC levels in the ocean rise, the capacity of seawater to absorb anthropogenic CO2 emissions will become lower. In addition, the oceanic carbon sink strengths may also change due to decadal variations and climate change scenarios.
Although ideas to increase carbon sequestration in oceans via the biological carbon pump by encouraging plankton growth (by adding nutrients like iron, nitrates and phosphates) have been proposed, not much is known about the unintended negative effects of this approach. So far, the increased CO2 levels in the oceans have already reduced seawater pH causing ‘ocean acidification’. Predictions of this trend indicate that by 2050, seawater pH may fall by 0.4–0.5 units to as low as 7.7–7.8. This acidification, coupled with global warming, increases the solubility of calcium carbonate in seawater and is severely impacting the survival of corals, shelled molluscs, and fish.
Read more: The Arabian Sea faces an increased frequency and duration of marine heatwave days, finds study
How do terrestrial systems act as carbon sinks?
Terrestrial systems – vegetation, soil, permafrost, and fossil fuel reserves – are important carbon reservoirs, though their carbon stocks are an order of magnitude lower than those of the oceans. Plant life fixes CO2 from the atmosphere through photosynthesis and traps carbon in organic molecules that are then stored in these reservoirs.
Forests have absorbed roughly 20–30% of the global CO2 emissions between 2001 and 2010. Between 2001 and 2019, forests have absorbed roughly 7.6 gigatons of CO2 per year; the forests in the Amazon and the Congo river basins have been the strongest carbon sinks during this time. However, the increased growth of forests due to higher CO2 levels, which causes trees to grow faster, may also shorten their lifespans. This means that forest carbon stocks may be more transient than expected, as trees will begin dying earlier, which will release the sequestered carbon back into the atmosphere.
In addition to forests, grasslands and peatlands are also considered important terrestrial carbon sinks. Both areas have the potential to absorb and store large amounts of carbon as living vegetation biomass and soil organic matter. At one time, managed grasslands were thought to function as perpetual carbon sinks; however, closer examination of the data indicates that this may not be so. Additionally, a study in 2021 suggests that the climate warming effects of managed grasslands may actually cancel the cooling effects of sparsely grazed natural grasslands that act as carbon sinks.
Peatlands are considered to be the most space-efficient terrestrial carbon storage systems since they produce more biomass than they lose, as the anaerobic conditions in their soils allow for low decomposition rates. Although peatlands form only 3% of the Earth’s land surface, their total carbon pool is twice that of the world’s forests; despite this, they are not taken into account in Earth Systems Models that predict future climate change scenarios. However, peatlands are poised to shift from being carbon sinks to becoming carbon sources due to global warming, peat wildfires, and draining of peatlands for peat extraction (peat is used as a fuel and in horticulture) and creating plantations.
How does India plan to use carbon sinks to offset carbon emissions?
Although there are several strategies to either create new carbon sinks (by using buildings, geological formations, or through direct CO2 capture techniques, etc.) and increase the carbon sequestration of existing sinks (via ocean fertilisation; conservation of forests, peatlands, and grasslands; forest regrowth; and soil carbon sequestration), the efficacies of these techniques are still unclear.
India’s forest carbon stocks, when estimated in 2004 by the Forest Survey of India, stood at 6663 million tonnes (6.6 Petagrams or 29.2 billion tonnes of CO2 equivalent). Currently, India’s NDC (nationally determined contributions) goal under the Paris Agreement is set at creating an additional 2.5–3 bilion tonnes of CO2e through additional forest and tree cover by 2030. According to a 2021 report from TERI (The Energy Research Institute), this will be possible only if India doubles its forest cover by 2030. The report highlights the importance of exploring blue carbon initiatives (carbon storage potential in coastal and marine ecosystems), integrated approaches to sustainable forest management, and the agroforestry industry to achieve the NDC goals.
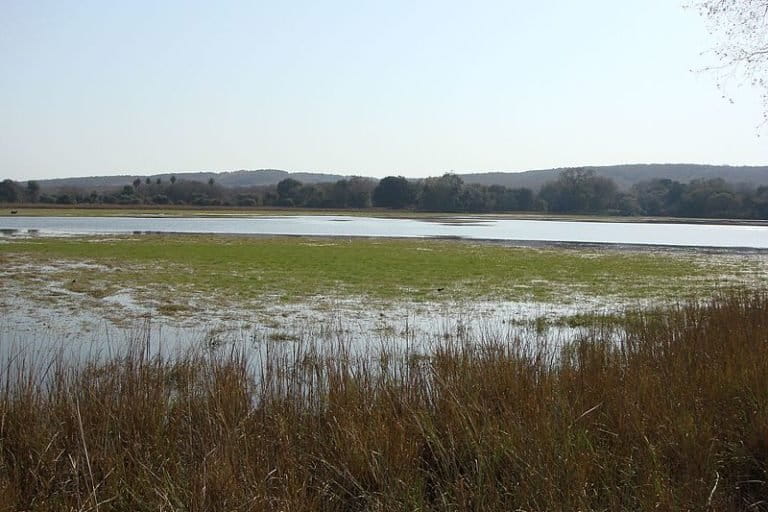
A study in 2021 which explored the potential of India’s agroforestry industry to act as a carbon sink estimates that a 30% expansion in the sector has the potential to offset the country’s carbon emissions by 2050. The research also indicates that agrosilvopastoral systems (which integrate the growth of crops, trees, and livestock) have the highest carbon sink capacities as compared to other systems such as agrisilvicultural (crops + trees) and silvopastoral (trees + livestock).
However, an older study in 2020 showed that species-rich natural forests are much better at carbon capture than the species-poor plantations that are created and managed in agroforestry. “Our study illustrates two ways in which biodiverse forests make better carbon sinks than monoculture plantations – these forests store more carbon in their vegetation and offer more stable rates of carbon capture over years, than plantations,” says Anand Osuri, a DBT Ramalingaswami Fellow with the Nature Conservation Foundation, and the lead author in this study.
“We found that teak and eucalyptus plantations – which were abandoned and not actively managed – not only store 30–50% less carbon than a wet evergreen forest, but the plantations’ ability to capture carbon from the atmosphere is more vulnerable to disturbances such as droughts. While we did not investigate the reasons for these differences in our study, one possible explanation from ecological theory is that high species diversity increases ecosystem productivity and resilience,” he adds.
Read more: What is carbon pricing?
Banner image: A haze of pollution seen over the Nalban water body at Salt Lake city, Kolkata. Photo by Biswarup Ganguly/ Wikimedia Commons